Highly Doped Silicon Substrates for OTFT Fabrication
A Ph.D. requested a quote for the following:
My Ph.D. work is majorly on making OTFT (Organic Thin Film Transistors) by testing various Solution Process-able Organic Semiconductor Inks on two kinds on highly doped Si substrates with Sio2 and Silicon Nitride (Si3N4) deposited on the top side.
Usage :
- I will be using bottom side high doped Si as the Gate electrode and top side " Sio2 or Si3N4 " as a dielectric.
- On top of Dielectric layer I will spin coat the organic semiconductor and on top of that I will deposit a gold metal by thermal evaporation.
Therefore I would like order very high quality
- (a) "Highly doped Si substrates with Sio2 deposited on the top side " and
- (b) "Highly doped Si substrates with, LPCVD Silicon Nitride (Si3N4) deposited on the top side.
Note: As I intent to use bottom surface of high doped silicon as gate, I would request for the Oxide on only one side of the substrate. That is the bottom dielectric should be fully etched as I dont want to do Buffer oxide etching by myself.
Therefore can you provided me the cotation for:
(1) 4" N-Type/As, [100], <0.005 Ohm-cm, 525+/-25um, Single side polished, prime grade, 2 SEMI Flats, 4500A (450 nm), 3000A (300 nm), 2000A (200nm) LPCVD Silicon Nitride (Si3N4)
(2) 4" N-Type/As, [100], <0.005 Ohm-cm, 525+/-25um, Single side polished, prime grade, 2 SEMI Flats, 4500A (450 nm), 3000A (300 nm), 2000A (200nm) SiO2.
Also, let me know, Is it possible to directly send me the diced substrates of the required dimensions.
Aside: As I am research student and if all goes well with quality and pricing, will be ordering the substrate for the next 3 years of my Ph.D. So I request you to please quote me the affordable rates.
Reference #225835 for specs and pricing.
What Are Organic Transistor Benefits?
An organic transistor is a semiconductor that uses an organic material for its channel. The advantages of
an organic transistor are several: it is flexible and inexpensive. Lightweight, and it has a high luminous efficiency. In this article, you will learn about three benefits of OLED. So, what are the other benefits of OLEDs? After reading this article, you will have a better understanding of these semiconductors. Here are a few:
What are OLEDs Luminous Efficiency?
Organic transistors have high luminous efficiency mainly due to their ability to suppress exciton quenching and photon loss. These transistors are made from an array of organic materials, including PMMA, Silicon, and Lithium Fluoride. Their high luminous efficiency makes them a promising candidate for LED applications. The following section provides an overview of the process used to fabricate an OLED. It will serve as a guide for designing an OLED.
The main optoelectrical properties of organic transistors are shown in Figure 2. The thickness of Ag in both devices is 80 nm. The intersystem crossing probability is 100%, which translates into a higher luminescence efficiency. The OLET is about 100 times more efficient than an OLED. The efficiency of organic transistors is also dependent on the amount of charge carrier transport in the device. The two devices have different threshold voltages and have high mobility.
Organic light-emitting transistors (OLEFETs) have the advantage of having a vertical charge transport. The in-plane charge transport is better compared to that of conventional LEDs. In addition, the higher electroluminescence quantum efficiency of OLETs is due to their device structure. The high luminous efficiency of OLEDs is expected to be useful in various applications, from wearable electronics to lighting.
OLETs represent an effective optoelectronic device platform that will enable novel organic photonic display and application technologies. As an OLET combines electrical switching with light emission, it is easy to process and does not produce pinholes or shorts between injecting contacts. Further, OLETs are very efficient and low-cost to produce. Therefore, organic transistors may be a viable alternative for lighting applications in the future.
What Do Organic Transistors Cost?
Organic transistors are extremely low-cost semiconductors that have many benefits. They are fundamental building blocks of digital combinational logic circuits and flip-flops. They are used in modern microprocessors, memory devices, and integrated circuits. They are also extremely flexible, allowing them to function as both a switch and an amplifier simultaneously. In this article, we will explore some of the most common applications of organic transistors.
A major advantage of organic semiconductors is their ability to be easily processed. The resulting silicon-free transistors are compatible with a variety of processing methods. One such example is an Organic Field-Emitter Transistor (OFET), which consists of a conducting polymer, which acts as both a source and a drain, on top of an insulating polymer made of polyester or phenylsilane.
In recent years, organic semiconductor printing has become increasingly advanced and scalable. Several techniques have been reported for the fabrication of organic field-effect transistors, which exhibit impressive mobility values. However, some of these techniques require additional crystallization steps. In this study, we report the fabrication of OFETs from four benchmark organic semiconductors and polystyrene. This method yields uniform and reproducible crystalline films.
High-density flexible sensor arrays are a necessary step for intelligent artificial skin and wearable electronics. Several researchers have devoted their research efforts to this goal, reporting fully-printed ten-by-six transistor arrays. In addition, they reported the fabrication of flexible and low-cost stretchable synaptic transistor arrays. They also developed a novel water-based silver nanowires screen-printed ink to make OFETs.
How Flexible are Organic Transistors?
Flexible electronics are the future of technology. Organic transistors, or OFETs, are flexible and low-cost semiconductors made from natural substances, such as sugars. The mobility of the carriers depends on the chemistry of conjugated frameworks and intermolecular p-orbital overlaps. Organic semiconductors are typically either organic molecules, oligomers, or conjugated polymers. Oligomers have inferior electrical characteristics and are difficult to process. However, conjugated polymers are relatively easy to process and have excellent flexibility. This article discusses the fundamental properties of flexible organic field-effect transistors, the technical challenges, and the potential applications for the technology.
The properties of organic transistors allow them to be fabricated in a wide variety of shapes and sizes, and are particularly versatile. For example, they can be embedded on banknotes, which can serve as the ultimate anticounterfeit technology. Another example is flexible sensors, which could be used to realize an electrically powered artificial skin. Likewise, flexible photodetectors could be implanted in the ear, which can detect and track movement. Since flexible OSITs have excellent mechanical flexibility, they can be implanted and wearable.
While silicon-based semiconductors are a great way to make electronics, flexible organic transistors are cheaper and more flexible. Many materials scientists are exploring the electrical and mechanical properties of bending thin-film electronic devices. Organic transistors can be made from biocompatible materials that are biocompatible. The result will be flexible, affordable, and biocompatible electronics. So, the future of flexible electronics may lie in flexible organic circuitry. Just keep in mind that these transistors are still in their infancy.
The performance of flexible FGTMs was demonstrated in a study by Zhou and co-workers. High carrier mobility was observed in a vertical device architecture. Additionally, the ultra-short channel length further reduced the effects of defects and ensured high operating speeds. The mechanical stability of these devices is superior to those of silicon-based floating-gate transistors and are within two orders of magnitude of the latter. So, they are a promising option for developing flexible memory systems.
Are Organic Transistors Used in Electronic Devices?
Organic transistor are a good choice for flexible electronic devices. Its light weight makes them ideal for such applications. They are easily fabricated on flexible plastics, which makes them extremely flexible and lightweight. The Que display, for example, is based on a 1 million-transistor array, which replaces the silicon-on-glass array. The Que's display has a flexible plastic backing and is virtually unbreakable.
Compared to inorganic semiconductors, organic semiconductors have more flexibility. Their molecular weights are smaller and they form conductive channels. However, unlike their inorganic counterparts, organic semiconductors have weak intermolecular bonds. They are held together by weak van der Waals forces. As a result, thin-film organic semiconductors are much more flexible than their inorganic counterparts. They can also be fabricated into flexible electronics.
The performance of organic transistors is inferior to silicon transistors. They exhibit poor electrical conductivity compared to silicon transistors. They can be produced at a much lower cost, but they have a shorter lifetime. One major advantage of organic transistors is that they are light in weight and flexible. Moreover, they are low in cost, and they can be made on a large surface area. The electrical properties of organic transistors and their performance in comparison to classical transistors are described in the paper.
The most common materials used in organic transistors are polymers. Plastics, such as polyethylene terephthalate and PEEK, are ideal materials for flexible electronics. Moreover, the polymer substrates are resistant to wear and tear. A polymer substrate can be used to make an organic transistor. The operating voltage of the organic transistor is low compared to silicon-based devices, which are heavy and bulky. This characteristic makes organic transistors a good choice for light-weight applications.
How Stable are Organic Transistors?
The structural and electrical instability of organic semiconductor field effect transistors has been observed in both metal insulator semiconductors and capacitors. This instability is attributed to the electronic trapping mechanism that takes place between the organic conjugated chain and the semiconductor. The oxidation of the organic semiconductor layer results in the formation of oxidation sites at these sites. This process is believed to lead to the formation of the trapping sites. The phase transition between the semiconductor layer and the metal insulator also causes this instability.
Various additives have been employed in the fabrication of organic transistors to improve their stability. The data in Fig. 2A demonstrates the highest stability achieved with sc-organic transistors, while the orange-dashed data shows the best stability with OTFTs. The results were consistent within an order of magnitude despite the difference in the experimental methods. The DVTH values observed by researchers in this study are also consistent with those obtained using a variety of organic semiconductor layers and different batches of devices.
In recent years, researchers have made major advances in the design of organic transistors. These devices exhibit good mechanical flexibility and are a promising candidate for flexible displays, robotic sensors, and biomedical electronic applications. One of the major challenges in the design of these devices is thermal instability. Due to their large thermal expansion coefficients and low melting temperatures, organic transistors suffer from thermal degradation. This instability is minimized by using flexible thin-film transistors that have exceptional thermal stability. These transistors are suited for biomedical sterilization processes and can be printed on various substrates, including flexible ones.
The fabricated devices were stored in uncontrolled environments for four weeks. Their temperature and humidity were recorded and their electrical properties were measured. The relative changes in mobility and threshold voltage were calculated using a model in which the gate dielectric capacitance is a function of the gate-dielectric properties. The results of the experiments showed that the electrical properties of organic transistors remain stable in ambient air and that the experimental conditions were reproducible.
What are Organic Field Effect Transistors (OFET)?
Basically, an organic field effect transistor (OFET) is a type of device that is made from an organic semiconductor that is used in a channel. These transistors have many interesting features and can be quite useful in many applications.
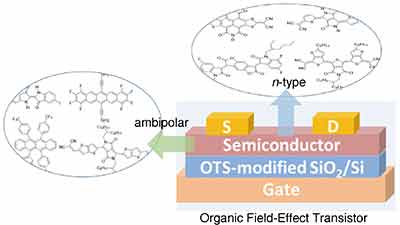
Basic electronic features
During the last few decades, organic field effect transistors (OFETs) have undergone a number of technological advances. They have achieved remarkable maturity and have a wide range of applications. These devices are made up of small molecule organic semiconductor single crystals. This review summarizes the basic electronic features of these devices. It also reviews the materials used in their fabrication and provides an outlook for future research.
In order to understand the working mechanisms of these devices, it is necessary to understand the transport of charge carriers. The first step is to understand the basic physical principles behind carrier injections at metal/semiconductor interfaces. Several mechanisms can be used to explain this process. Among them, the Mott-Schottky rule is an important one. This rule states that electrons can be injected into polycrystalline tetracene thin films. It is based on the concept that most charge carriers do not leave the device at the base electrode.
The next step is to understand the carrier transport in soft semiconductor crystals. This is of increasing importance for organic flexible electronics. Organic single crystals are the best candidates for an ideal carrier transport system. They have intrinsic charge dynamics, enabling us to study the physics of charge transport in molecular semiconductor systems.
The most common materials used in OFETs are pentacene and rubrene. These materials have been widely studied over the past few decades. Pentacene has been the most common material for OFETs, but it is also harder to fabricate because of its chemical instability with oxygen in the atmosphere. Rubrene has achieved a benchmark carrier mobility of 20-40 cm 2 Vs -1.
A variety of materials can be used for SC-OFETs. Thin platelets of rubrene single crystals can be used to achieve the benchmark carrier mobility. This material is prone to disorder, which can decrease mobility. Thin base electrodes are also important for these devices. These devices have a large field-effect response, but small pores may form in the layer.
The FI-ESR signal originated from field-induced carriers. In addition to the carrier mobility, this signal also consists of spin numbers. These spin numbers agree well with the gate capacitance.
Typical OSC-2D heterojunction structure
Typical OSC-2D heterojunction structure can be used for different opto-electronic functions. The performance of a heterojunction can be affected by the band alignment at the interface. Band alignment can be defined as the spatial separation of electrons and holes. The band structure can be controlled by a light or magnetic field. It is the basis of most of the illuminance properties of 2D heterostructures.
The band alignment can be classified into type I, type II, and type III. Type I band alignment is asymmetric, which means that the holes and electrons are arranged in staggered orientations. It can improve the exciton efficiency and is suitable for photoluminescence detections. In addition, type I heterojunctions can prevent undesirable dissociation of excitons.
Type II band alignment involves a spatial separation of holes and electrons. It is commonly found in TMD heterojunctions. It can be used to build vdW Esaki tunnel diodes. This type of heterojunction is also used to regulate valley pseudospin in 2D materials. In addition, type II heterojunctions can generate a strong built-in electric field.
Type III band alignment is much faster than type II band alignment. It can be used to build vdW diodes with a wide bandgap. It can also be used to generate tunnel field effect transistors. The bandgap of type III heterojunctions can be tuned by changing the thickness of the heterojunction. This type of heterojunction is also referred to as a broken bandgap.
In addition, dangling covalent bonds are an inherent property of organic semiconductors. They allow for trap free surface layers and reduce lattice mismatch. They also enhance absorption at longer wavelengths. These properties are useful for designing biocompatible devices.
In addition to these properties, graphene is also gate-modulable. It has unique linear dispersion relationship and can be used to reduce contact resistance. In addition, it has strong Fermi-level pinning at defects. It is widely used for photovoltaic applications. The bandgap can be adjusted from 0.3 to 1.8 eV.
In addition, a new sandwich stacking vdW structure has been proposed to realize prominent performance photodetectors. The benefits of this structure include strong coupling, efficient charge transfer, and high speed.
Electrode materials for OFETs
Various electrode materials have been developed for Organic Field Effect Transistors (OFETs). The material systems include conjugated p-electron systems, electron withdrawing groups, electron donating groups, and aromatic p-electron systems. Each has unique properties, and the carrier mobility of OFETs depends on their chemical structures. In addition, the nature of the interface is also important to the performance of OFETs.
The first organic field effect transistor was developed by the researchers at Mitsubishi Electric in 1986. This device was based on a polymer of thiophene molecules. It was a highly promising device for low-cost mass production. In the following years, researchers began to explore the development of organic semiconductors. The advantages of these semiconductors were their high flexibility, low-temperature processability, and good sensitivity.
Compared to conventional transistors, OFETs have a smaller operating voltage, lower power consumption, and high sensitivity. They also have the advantage of being able to work in aqueous environments. However, these semiconductors are not as well-suited for applications in flexible electronic devices. However, they have received a lot of attention. They have recently achieved remarkable progress in the field of integrated circuits.
These devices have been designed to be able to sense a variety of biological signals. These include blood pressure, nerve impulses, and cell control. They also have the potential for monitoring human activity and personal healthcare.
Recent advances in the interfaces for OFETs have led to the development of organic light-emitter transistors. In addition, conformable sensors have the potential to be used as human-machine interfaces. However, they have yet to be developed in sufficient scale. There is a need to explore the integration of OFETs into more complicated integrated systems.
An additional functional receptor can increase the sensitivity of an OFET-based sensor. In addition, conformal contact is dependent on the stiffness of the sensing device. In addition, the adhesion energy of the sensing device is also important to the performance.
Among various electrode materials, carbon-based nanomaterials, such as graphene and carbon nanotubes, have a unique advantage in that they exhibit excellent thermal stability. They also show excellent electrical conductivity. However, their demerits include low mobility and poor grain boundaries.
High photoluminescence emission and photoluminescence quantum yield
Using a centrifugal coating technique, CsPb 2 Br 5 NPLs were produced in NPL-LEDs with PLQY of 35%. These NPL-LEDs showed maximum luminance of 41.8 cd m -2, which corresponds to CIE color coordinates of 0.09 and 0.78.
The PE of these NPL-LEDs was 0.4 lm/W. In addition, the average EQE was 21.3%, which was significantly higher than that of the CsPbBr 3 NPLs.
The graded shell NPL-LEDs showed a steep increase in EQE and luminance behavior. In addition, the EQE increased to 0.5% for sky blue (489 nm) LEDs. These NPL-LEDs also showed a steep increase in maximum luminance, with an average FWHM of 16 nm.
In the crystalline state, herringbone stacking modes are commonly observed in phenylene/thiophene derivatives. These modes can balance charge mobility in the crystalline state, and help to achieve high fluorescence quantum yield.
For these NPL-LEDs, the ligands were replaced with 3-mercaptopropionic acid, which enhanced charge injection. The use of a thin CBP layer as a hole injecting layer also helped to improve charge balance. The use of 3-mercaptopropionic acid resulted in an increase in EQE of 0.63%.
Moreover, the thin CBP layer was used as an HTL between CsPbBr 3 NPLs and poly-TPD HTL. This ligand exchange was performed to increase the fluorescence quantum yield of the phosphorescent LEDs. The fluorescence quantum yield was lowered by 40% after the ligand exchange. In addition, the NPL-LEDs had a turn on voltage of 3.8 V.
The EL emission spectra of the NPL-LEDs were measured at different voltages. The emission spectrum could be tuned by varying the ratio of Se to S. This could be used as a method to continuously adjust the emission spectrum. The EL spectrum was adapted with permission from the American Chemical Society.
The EQE of the CdSe core/crown NPLs was also studied. It was found that the PLQY decreased from 37% to 10% as more sulfur was alloyed. The EQE of the CdSe alloyed component was also lower, because less passivated dangling bonds were formed in the CdS component.
The relative rates of intersystem crossings may determine the final property. The higher the excitation density, the larger the photon echo, which will lead to an accumulated photon echo. These results suggest that the fluorescence quantum yield of the phosophores may be enhanced by using an efficient fluorescence quenching process.