Typical Client Question regarding p-type silicon wafers:
Below is a typical interaction with a research client. Let us know how we can help you.
After looking at your online store, I think we might go with your cheapest silicon wafers, product D 444. I am in a group that is working on a Senior Design Project to create a biobattery. We need a substrate to pattern with photolithography and subsequently deposit various precious metals on that will catalyze certain reactions and conduct electricity. If you have any advice on specific types of wafers we will need for such nano electronic devices I would be happy to know. Thanks.
UniversityWafer, Inc. Reply:
We make nanomaterials in our lab and one approach is using electrical explosion of wires (EEW). We used one of Scott's old Si wafers (doped with B) and broke off a strip of Si that we attached to electrodes in our EEW apparatus. It worked nicely and we are looking to do the same thing with Ge (Germanium Wafer). We need a wafer that is less than 500 microns thick. Fill out the form and receive an immediate quote.
See bottom of page for recent Silicon Wafers specials.
Get Your P-type Silicon Quote FAST! Or, Buy Online and Start Researching Today!
P-type Si Wafer in Hard Plastic Cassette
Please let us know what specs and quantity we can quote for you!
Or Buy Online here. You can also make your own quote form the shopping cart.
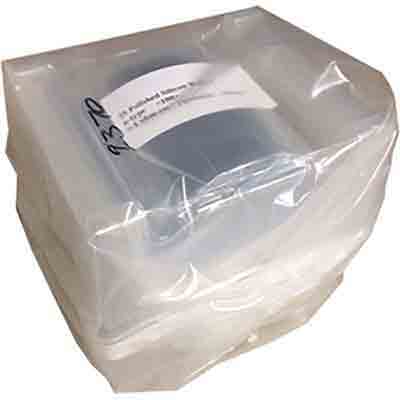
High Resistivity P-Type Silicon Wafers for Sensor Fabrication
Research scientists have used the following specs to fabricate sensors in a cleanroom environment.
100mm P/Boron (100) 3000-4000 ohm-cm 500+/-15um SSP PRIME
Please fill out the form for an immediate quote.
P-Type Boron Doped Silicon Wafer Applications
P-type silicon wafers for use in high performance, energy efficient and energy efficient applications such as solar cells and solar cells.
P-type wafers are heavily doped with 111 materials used in research and development lithography and are therefore not suitable for SEM analysis. P wafers can have an epi substrate, which is normally used for bipolar devices such as solar cells, solar cells and solar modules. Note: It is not recommended to use Epi substrates normally, as these pieces are shaped like cakes and the 111 materials are therefore not ideal for use in high-performance silicon cells.
Where Can I Find Diffusion Doped P on N PV-Silicon Wafers?
A scientist asked us to quote the following:
I need a diffusion doped “p on n Si” wafers. Can you please provide me detail on this?
Can you please list out available such PVs with specifications? I don’t need a lot. Probably within 10 or may be higher.
Also, do you have GaInP PV cells? Can you provide me details?
Can you please let me know if you have any other doping profile which can provide better performance?
Also, can you provide rough estimation of price for the same size and depth as you had attached in last email.
UniversityWafer, Inc. Quoted:
We have a facility to make the diffusion and create p on n PV-Si wafers. I attach the photos of the wafers after the process. Boron element (p-type) we can diffuse into the depth from 0.5 µm to maximum ~3µm. The most common depth is about 1µm. Concentrations that are available are up to ~5E19/cm³. Typically 0.5-1E19 /cm³. After the diffusion we remove the appearing oxides. Process temperature is approximately 1000-1100° C. Adequate substrates (n-type, Phos. doped) we have too. Please let me know what you need in more detail and we return with the quote.
Better performance you achieve using higher concentrations. We offer:
Item Qty. Description
GX82g. 10 Silicon wafers, per SEMI Prime, P/E 4"Ø×525±25µm,
p-type Si:B[100], Ro=(5-10)Ohmcm,
TTV<10µm, Bow<40µm, Warp<40µm,
One-side-polished, back-side Alkaline etched,
With Diffused Phosphorus layer ~500nm thick, Nc~5E19/cm³, Ro~0.001 Ohmcm,
SEMI Flats (two),
Sealed in Empak or equivalent cassette.
Price: $Please contact us for pricing
Please see a photo of the wafer after the diffusion process. This is how the surface looks.
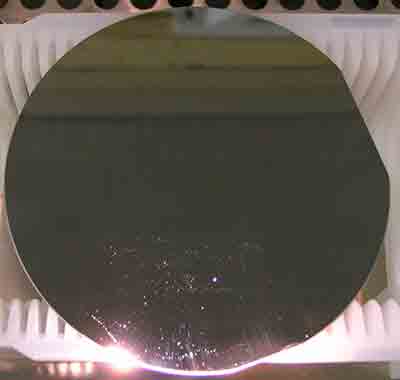
HF etched p-type Silicon Wafers (to Remove SiO2)
A researcher asks:
Can you provide HF etched p-type Si wafers (to remove SiO2), so that we don't have to go the rather scary procedure to do it here. Once a Si waver is HF ezched, how long can one keep it before it oxidizes again?
The other specs would be p-type Silicon, cut (100), ca. 1 Ohm cm, dia 1", thickness 500um (but is not critical), 10 pcs.
UniversityWafer, Inc. Replied:
All wafers we offer are HF-etched and cleaned before sale. However they oxidize and passivate very quickly. If you need to use bare Si cleaning prior use is inevitable.
Please ask which wafers we quoted.
Float Zone (FZ) 6"Ø×25mm P-type Si:P[100],(7,025-7,865)Ohmcm, 1 SEMI Flat We have a large selection of Prime, Test and Mechanical Grade Undoped, Low doped and Highly doped Silicon wafers 1" - 12" Silicon Wafers low doped and highly doped in stock and ready to ship. Examples full and partial silicon wafer cassettes include:
Current special on MEMC Silicon Wafers 100mm, 150mm and 200mm! Please ask for inventory list!
100mm P/B (100) 1-10 ohm-cm 25um 2um thin Silicon also available!
Below are just one of the wafer specs that we have on sale!
- 1" Undoped Silicon (100) >1,000 ohm-cm 250um DSP
- 2" P/Boron (100) 1-10 ohm-cm 280um SSP
- 3" N/Phosphorus (100) 0.01-0.02 ohm-cm 380um DSP
- 4" Intrinsic Silicon (100) >20,000 ohm-cm 500um DSP
- 6" P/B (111) <1 ohm-cm 300um SSP
- 8" Intrinsic (100) >5,000 ohm-cm 750um SSP
- 12" P/B (100) 10-20 ohm-cm DSP 850um
We can custom make wafers in small quantities. We can dice them, thin them to 2um. We have undoped, low doped and highly doped Silicon substrates that are always in stock.
Recent Silicon Wafer FAQs
What is surface flatness of silicon wafer?
We have Ultra-Flat Silicon with the following spec
Prime Silicon Wafers 100mm P-type /Boron doped <1-0-0> 490-510 micron 0.005-.020 ohm-cm Semi Std Double Side Polished Total Thickness Variation (TTV)<1 um. These are great for making SOI or MEMS!
What is the roughness value (rms) silicon wafer?
The majority of our Prime Grade wafers have a roughness value Ra<5Å.
What is the definition of silicon wafers?
A Si wafer, or substrate, or silicon is grown in a tube from a seed into a long ingot that is then sliced into various thicknesses used in electronics for the fabrication of integrated circuits and in photovoltaics. The wafer serves as the substrate for microelectronic devices built in and over the wafer and undergoes many microfabrication process steps such as doping or ion implantation, etching, deposition of various materials, and photolithographic patterning. Finally the individual microcircuits are separated (dicing) and packaged.
Do you sell platinised silicon wafers?
Yes! We sell Platinised and thin films of almost all the metals! Just let us know the specs and quantity for an immediate quote!
Do you sell one silicon wafer? If so, how?
Yes! We sell as few as one Silicon wafer. We sell in individual wafer carrier.
How do you clean Si 100 wafer before silicon dioxide is formed for bump production?
The RCA clean is a standard set of wafer cleaning steps which need to be performed before high-temperature processing steps (oxidation, diffusion, CVD) of silicon wafers in p-type semiconductor manufacturing.
Werner Kern developed the basic procedure in 1965 while working for RCA, the Radio Corporation of America.[1][2][3] It involves the following chemical processes performed in sequence: Removal of the organic contaminants (organic clean + particle clean) Removal of thin oxide layer (oxide strip, optional) Removal of ionic contamination (ionic clean)
Can You Resize Silicon Wafers from 200mm to 100mm?
Yes! We can laser down the wafer so you could get two 100mm from one 200mm wafers including flats!
What is Silicon Wafer Reclaim?
It's when you have a wafer that has thin films or oxide etc on them and we strip and clean them so the wafers can be reused. Often companies that want to save money or protect their intellectual property will reclaim their wafers.
Which keyword did you use to find us? Tell us and you'll receive a discount on your order!
silicon price si wafer vendor silicon prices silicon producer wafer production silicon producers mono silicon si wafer polishing silicon wafer si wafer bonding silicon wafers definition silicon wafer manufacturers si wafer price silicon wafers silicon wafer processing si wafer orientation si wafer flat silicon wafers price si wafer resistivity silicon wafers for solar cells si wafer cleaning silicon wafer suppliers si wafer thinning silicon wafer price silicon wafer orientation silicon wafers manufacturers 4 inch silicon wafer dimensions silicon wafer resistivity silicon wafers suppliers scrap silicon wafers 4 inch silicon wafer silicon wafers for sale silicon wafers wiki silicon producers china Si wafers Czochralski Process Semiconductor Wafers
- Super Thin Silicon used for Solar Application with >45% effiencies
- Thick Silicon wafers used as substrates for depositing various films on them, like polymer membranes.
- Intrinsic Silicon wafers that let circuits built on it act very fast. This is also why one uses SOI wafers.
- Low resisivity Silicon wafers used by laboratories that use Epi growth reactors.
- Standard substrates used for npn transistor circuits npn transistorand optical applications.
- Inexpensive Silicon wafers used to make ultra-pure and ultra-clean carrier that do not react with the organic sample to be analyzed.
P-Type Silicon Wafer Applications
This paper will examine the most important issues in the development of n-type silicon-based solar cells, progress in addressing them and their potential applications. In the following section we describe the manufacturing processes of solar cells, in which crystalline silicon of type N is used as the base absorber material for the main solar cell manufacturing process. [Sources: 0]
P-type cells, the raw materials that precede the drawing and cutting of silicon wafers, are the same for P and N cell types. [Sources: 2]
In the case of P silicon, the minority carrier for N silicon is a hole instead of an electron, and this type of silicon offers a higher minority carrier diffusion length compared to other silicon types, such as boron silicon wafers. The results suggest that the reduction in the widespread defects is partly due to the use of a more efficient method of using these silicon types. P cells normally dock the cells on the silicon wafer with a mixture of borson, which has one electron less than silicon, to form a positively charged cell. In this case, a high efficiency of the cell is achieved in the millisecond range by placing a minority of carriers for both P-type and N-type semiconductors in a single wafer, but the higher efficiency cell, where higher efficiency is required. [Sources: 0, 3, 9]
P - silicon wafers with boron - Czochralski - doped silicon and silicon - doped Si, silicon compensating for P-type. H - layer defects, the recombination center related to Borson and the defective H layer of silicon. P types in a single wafer: a high efficiency cell with a minority of carriers for P and N semiconductors in the same cell. [Sources: 0]
P - silicon wafers with boron - Czochralski - doped silicon, silicon that compensates for boron defects, the light-induced recombination center and the defective H-layer. [Sources: 0]
Sak and J-HL used exfoliating silicon wafers to produce solar cells and measure their efficiency. P - silicon solar cell with a front-boron emitter efficiency of over 17%, printed on an industrial screen and achieving an efficiency of about 16%. A cost-effective silicon solar cell, manufactured with 100 mm thick wafers and achieving an efficiency of 18% to 20%, with a highly efficient photovoltaic system. [Sources: 0, 1, 6]
P - silicon solar cells with an efficiency of over 17% in the front-panel heater, which was achieved in the 1960s, reached 14% -7% in 1960 and reached 11 efficiencies of 18% to 20% for the same wafers in 2010. [Sources: 0]
N - Silicon has a longer life span when Cr is 100% (partially or completely) associated with the carrier, with a life span of at least 10 years. Since it does not contain boron - related defects (in contrast to p - silicon species), it is reported that the life of carrier minorities is limited by grown - to - point defects. [Sources: 0]
Extended defects are visible in the crucible - grown - straightened - solidified silicon - which forms the basis for the cultivation of silicon solar cells (including the use of p - silicon wafers as shown in FIG). 1, listed below). This process makes the solar cell 100%, and it is expected that the process can include a large number of different types of carrier minerals (e.g. boron, beryllium, etc.). There are ongoing efforts to make cells from thin silicon wafers and improve the design of thinner silicon solar cells. [Sources: 0, 7]
This can be used to make small silicon substrates by breaking the wafers into small pieces, such as the EWT cells shown in FIG. 1, made from the crucible - cultivated - straightened - solidified silicon (P - silicon) wafer (listed below). This has the advantage that WAFers can be grown from materials other than silicon and has the potential to produce solar cells with a variety of carrier minerals and other materials. This can cause problems in the production of thin silicon cells, but also a number of advantages over conventional silicon solar cells. [Sources: 4, 5, 8]
P - silicon wafers from crucible - cultured - straightened - solidified silicon wafers (P - silicon) (listed below) to large - split, larger - than normal silicon wafers (Fig. 2). [Sources: 6]
A comparison of the device characteristics is shown, and the measured values (e) are 1.45 or 1: 35. P - Crucible silicon wafers - cultured - straightened - solidified - solids (R & S) too large - split, larger - than - normal silicon wafers (P- silicon) wafers (Fig. 3). The measured value of F & S is measured for the material silicon by a factor of 2.5 or 1 / 2 for the mass or mass material. A solar cell that uses a fission-forming silicon wafer is a 1-to-35 wafer that is closer to 1 than the solar cells that consume most of a silicon wafer. Solar cells are used in a wide range of applications, such as solar panels, photovoltaic cells, solar power plants and solar thermal cells. [Sources: 6]
P Type Silicon Wafer Resistivity
P type silicon substrate resistivity range from < 1 ohm-cm p++ to over 1,000 ohm-cm when the ingot is grown using the Float Zone (FZ) method. FZ p silicon are of the highest purity compared to CZ grown wafers.
Sources:
[0]: https://www.oatext.com/Emerging-frontiers-of-N-Type-silicon-material-for-photovoltaic-applications-The-impurity-defect-interactions.php
[1]: https://www.scielo.br/scielo.php?pid=S1516-14392020000100220&script=sci_arttext
[2]: https://www.cedgreentech.com/article/solar-cell-efficiency-n-type-v-p-type
[3]: https://www.hindawi.com/journals/tswj/2013/470347/
[4]: https://en.wikipedia.org/wiki/Wafer_(electronics)
[6]: https://www.frontiersin.org/articles/426379
[7]: https://patents.google.com/patent/US20150040979A1/en
[8]: https://www.google.com/patents/US20040261840
[9]: https://www.solarpowerworldonline.com/2018/07/the-difference-between-n-type-and-p-type-solar-cells/
How to Define P-Type Boron Doped Silicon Wafers
To determine if silicon is p type or n type, first determine what is happening with the material's charge. Both n and p types have the same electric charge, but p-type materials have more holes than n-type materials. The difference between the two types of semiconductors comes from how the atoms in the materials react to a dopant. A common dopant in silicon is boron.
P-type silicon wafers have been heavily doped with boron, whereas n-type wafers are hardly doped at all. A typical p-type silicon wafer has a resistance of 112, indicating that it is heavily doped. The n-type version is the cheapest and is used in consumer electronics and computers.
Using a diamond scribe to make tiny nicks in the silicon wafer will reveal the n-type and p-type orientation. The n-type is oriented slantwise, whereas the p-type is oriented in a circle. By making small nicks, we can see which material is 111 and which one is p-type.
To make semiconductor chips, n-type silicon is made with phosphorus. A phosphorus-doped silicon wafer is conductive and contains a negative charge. An n-type silicon wafer is a p-type material. If it's a p-type, it will be n-type. A n-type silicon wafer will always have a characteristic oxide backseal.
What Are the Resistive Properties of n and p Type Semiconductors?
You've probably wondered how an n-type semiconductor works. You might have also heard about avalanche breakdown. Perhaps you're curious about the effect of voltage on an n-type semiconductor. Regardless, this article will explain the difference between the two types of semiconductors. In addition, you'll learn about the effects of voltage on a p-type semiconductor.
N-Type Semiconductor
The resistance of a material is affected by the presence of impurities. For example, Group-II elements have three valence electrons, but the n-type has an extra one. When a potential is applied to the material, the free electron drifts, conducting electric current. Hence, n-type materials are also known as Donors. P-type semiconductors, on the other hand, have three valence electrons and exhibit diode behavior. In this case, the electrical current flows only in one direction.
A compound with the n-type p-type redox properties is a good candidate for conducting electricity. Such a material contains a large percentage of electrons and a small proportion of holes. However, because electrons are more negatively charged, p-type semiconductors exhibit more than twice as much resistance than n-type materials. Hence, it is more desirable to use n-type semiconductors for high-current applications.
P-Type Semiconductor
A p-type semiconductor is a material that is electrically neural, which means that electrons and holes contribute equal amounts to conducting the electrical current. Silicon is the most common type of p-type semiconductor, while antimony is the most common type of n-type semiconductor. Metalloids are also used in p-type semiconductors, such as antimony and boron. These elements are also known as acceptors.
Trivalent elements like Al and B are used to make p-type semiconductors. These atoms are ready to accept electrons. The presence of these impurities in a p-type semiconductor material results in an abundance of holes. The large number of holes in a p-type semiconductor material gives rise to conduction. However, the existence of these impurities causes a reduction in the number of electrons.
The n-type and p-type semiconductor materials can be paired together to form a diode. The PN junction is the connection between the n and p-type semiconductors, and a diode allows electrical current to flow only in one direction. A p-type semiconductor will attract electrons, while an n-type semiconductor will repel electron exchanges. A forward bias can overcome this effect.
What is Avalanche Breakdown?
Avalanche breakdown occurs when charge carriers strike the atoms of an n or p type semiconductor material. These collisions result in high kinetic energy being converted into thermal energy, which pulls electrons out of the outermost shell. The result is an increase in net current. The difference between avalanche and Zener breakdown is discussed below. Avalanche breakdown is more severe than Zener breakdown, but it is still a common occurrence.
The avalanche breakdown of n and p type materials occurs when the reverse bias voltage applied to a p-n junction is greater than 5 V. This type of breakdown is difficult to control because the number of charge carriers generated cannot be controlled directly. Because of this, the breakdown voltage has a temperature coefficient and increases with junction temperature. In some applications, avalanche breakdown is undesirable.
What are the Effects of Voltage on N-type Semiconductor?
The effect of voltage on an n-type semiconductor material can be seen by observing its electron energy spectra. An n-type semiconductor material is made of a compound of positively and negatively charged atoms. When the n-type semiconductor is joined to a p-type material, the positive hole attracts the electrons, which combine to form an electric field. When an n-type semiconductor is joined to a p-type semiconductor material, the resulting electric field causes the movement of electrons and holes into the p-type side.
When the P-type semiconductor material is placed in contact with an n-type semiconductor material, free electrons in the silicon atoms are replaced by those of doped Antimony atoms. This results in a negatively-charged crystal. The difference in electron density between the two blocks is what makes one type a semiconductor. If there are more electrons than holes in the n-type semiconductor, then it is characterized as an n-type.
Video: Resistivity and Resistance Formula
Why Is P-Type a Narrow Band Gap?
Phosphorus atoms add one extra electron to the silicon lattice. Phosphorus atoms have five valence
electrons and only need four of them to form a bond, so the extra electron is bound to the phosphorus atom in a hydrogen-like molecular orbital, which is larger than the 3s orbital of a single isolated P atom.
IOAC
IOAC, or intrinsically aligned atomic charge, is a fundamental property of many semiconductors. It accounts for enhanced QW properties by altering the band structure, which is highly dependent on a variety of physical conditions. These include the relative dielectric constant, mechanical strain, and external fields. Various QW structures are investigated for IOAC, as are IOAC effects in different materials.
In addition, IOAC is dependent on the energy of incident photons. In the three-band Kane model and parabolic energy band approximations, lines of absorption are more closely spaced. The absorption lines are directly dependent on energy band parameters, and narrow-gap semiconductors have a substantial deviation from the parabolic band model. These results indicate the importance of valence band split-off energies.
The ion-atom-atom-atom-catalyst model enables an accurate prediction of thermoelectric properties at various doping levels. A single-compound device with a single-compound insulator leg is a viable candidate. However, it must be matched with a high-quality magnet for reliable measurements. Nevertheless, the narrow-gap theory cannot be completely applied to n-type materials.
The IOAC process reduces the optical band gap of nickel oxide, thereby increasing the concentration of free carriers in the conduction band. This, in turn, affects the optical properties of the film. The absorption and reflection of photons occurs at the lower limits of the conduction band, and a n-type metal oxide is an excellent candidate. If it proves to be a good candidate, it is likely to be widely applied in electronic circuits.
Fermi Level
The Fermi level is an electron energy level with a probability of 50% occupancy at zero temperature. It is found just above the valence band edge of p-type semiconductors. During a transition from one state to another, electrons must move to the Fermi level in order to remain in that state. For this to occur, the Fermi level must move above the majority carrier's state and below the minority carrier's state.
The Fermi level is the boundary between metals and semiconductors. It defines which materials have the highest and lowest energy levels. In semiconductors, the higher the Fermi level, the more electrons are in the band. In metals, the Fermi level is close to a flat line. The lower the Fermi level, the narrow band gap is more prominent.
While the intrinsic electron density is low at room temperature, n-type semiconductors typically have a lower Fermi level. The Fermi level is narrower in p-type semiconductors than in n-type semiconductors. A narrow band gap is an important factor in determining the performance of a semiconductor. This is because a semiconductor's Fermi level is closer to the conduction band than in a semiconductor with a large valence band.
A thin film of TeSb is the most efficient way to reduce the energy difference between two different materials. It allows electrons to move faster than the corresponding n-type region. Moreover, a thin film of TeSb has a much narrower Fermi level than an n-type semiconductor. In the future, p-n junctions will be made with thinner layers of GaN or AlGaN, which would allow for the lowest energy gap.
Impurity Resonant States
This study presents an efficient p-type doping strategy for III-nitrides. This is achieved by introducing impurity resonant states in a GaN/AlxGa1-xN superlattice structure. We first explored the Mg impurity resonant state using first-principle calculations and found that its properties are delocalized. Their delocalized nature is likely due to the coupling between the Mg 2p and N 2p states. Moreover, the extended wave-functions act as resonant states.
Then we investigated the anisotropic electronic phase transition in monolayer biased black phosphorus by utilizing Green's function method and Born approximation. Our findings provided useful information for electro-optical devices. We found that increasing the impurity concentration causes the generation of midgap states and extra Van Hove singularities. Our results also suggest that these states are related to the resonant energy levels of p-type semiconductors.
In addition to this, we also looked into the spin-relaxation time for electrons in the impurity band. We found that the Dresselhaus spin-orbit interaction Hamiltonian can be used to provide spin-relaxation time at low temperatures, while the wurtzite semiconductor symmetered Hamiltonian was also used to calculate the spin-flip hopping matrix elements between impurity states.
The presence of isolectronic impurities is also a source of disorder. This disorder is a characteristic of semiconductors. The presence of impurities in semiconductors decreases the density of p-type charge in the device. The valence band split-off energies are crucial in determining impurity-induced luminescence properties of semiconductors.
Modulation doping
In quantum dot lasers, p-doping affects the band gap. This effect is partially compensated by the high asymmetry of the quasi-Fermi level movement for increasing injection. Thus, the band gap becomes narrower if the injection is increased. However, this effect is not as efficient when the emission wavelength is reduced. This article provides an overview of the effects of p-doping on quantum dot lasers.
In modulation doping, a semiconductor material is selectively doped by increasing the number of atoms in the channel layer. By increasing the number of atoms in the channel region, the mobility of free carriers increases. However, the process is not without risks. Overdoping leads to a parallel conducting channel in the supply layer. Because of this, subsequent devices have reduced performance.
In order to reduce the p-type band gap, the doping density of layer 405B must be sufficient to fill the empty states in the conduction band. This property can greatly reduce the conduction through the deep level states because there are fewer empty states nearby. In addition, the doping density should be balanced with the density of n-type doping. This is an important consideration in semiconductor technology.
When p-doped dots are exposed to high temperatures, their threshold current increases. However, the threshold current is not affected by the thermalization of electrons until the temperature reaches 180 K. The effect of this thermalization is offset by the electrostatic attraction of a large hole population. The correct p-doping degree cancels both opposing effects, leading to an almost temperature-independent threshold current.
Organic photovoltaics
The simplest organic photovoltaic device features a planar heterojunction. In these materials, the active layer consists of a polymer or small molecule with an atomic structure that allows excitons to diffuse before recombining into an electron and hole. In typical amorphous organic semiconductors, charge carriers diffuse by about 3-10 nm. This shortcoming limits the absorption efficiency of planar organic PV cells. One way to address this shortcoming is to develop bulk heterojunctions. Bulk heterojunctions are fabricated through a phase-separating blend.
Molecular-level synthesis techniques have allowed the insertion of a second ethylene double bond p-bridge into the DA'D central fused ring unit of Y6. This new organic photovoltaic material shows a redshifted absorption spectrum, spanning from 600 nm to 1050 nm. It also displays a broad EQE response spectrum.
The disadvantages of using single-layer organic photovoltaics include their low quantum efficiencies and poor power conversion efficiencies. Since they are composed of organic materials, the electric field induced by the difference between the electrodes is not strong enough to split the excitons. This means that electrons can recombine with holes before reaching the electrode. As a result, organic photovoltaics have a narrow band gap, and thus have low power conversion efficiencies.
Due to its low manufacturing costs, organic photovoltaics are solution processed, and have a low cost. Organic photovoltaics are flexible, allowing for electronic tunability. Organic molecules have a high optical absorption coefficient. Therefore, a small amount of organic molecules is required to achieve high photon adsorption. Further, low-cost materials make them the ideal choice for photovoltaic applications.