Silicon Wafers Used for Photodiode Processing
A postdoc requested a quote for the following.
I was wondering if you sold wafers used for solar cell or photodiode processing. That is, wafers already doped which we would just have to metallize and cut.
I am looking for a 3" SOI wafer. Epi layer p-type (boron), resistivity 1-5 ohm, <100>, thickness of 1 micron or less. Burried oxide layer 0.5 to 1 micron, and P-type substrate, <100>, resistivity 1-5 Ohm.
Question:
Also, we were looking for the buried oxide to be a SIMOX process, by ion implantation. Is this possible?
UniversityWafer, Inc. Answered:
Yes! We have!
Reference #140678 for specs and pricing.
Get Your Quote FAST! Or, Buy Online and Start Researching Today!
Silicon Segmented Photodiode
A senior research fellow working on a satellite requested a quote for the following.
I am a senior research fellow. Ccurrently my lab is in the initial phases of mission study for a mini
satellite (~ 250kg, Remote Sensing Applications) with highly demanding
requirements for satellite pointing and stability.
In this regard we are interested to develop two-axis sun sensors (using
quadrant sensor elements or triangle shaped sensing elements).
Below are the specifications for Si segmented photodiode.
Purchase Information:
Quantity Required: 10
Application: For satellite/space sun sensor development
Description: 4 Quadrant Segment Position Sensitive Detector (PSD)
No. of Element per piece: 4
Active Area / Element: 10mm x 10mm
Center Pitch: 0.005 mm
Photodetector Electro-Optical Specification Per Element:
Die Size: 25mm x 25mm
Active Area Size: 20mm x 20mm
Operating Wavelength: 200nm ~ 1100nm
Applied Bias: negative
Max. Dark Current @ ~ < 3nA
Max. Capacitance @ ~ < 3000pF
Mechanical / Packaging Specifications:
Isolated Chip: Yes
Common Anode or Cathode: Common Anode
Chip Only: with Solderable Pads
Environmental Requirements:
Space Environment: Low Earth Orbit
Temperature: -40°C ~ 75°C
Question:
We are interested
to find out whether you are manufacturing wafers that will help to build
our sensors. If you have such wafers for sun sensor design please provide
us the technical and commercial details so that we can initiate
procurement procedures.
UniversityWafer, Inc. Answer:
For the Sun-Sensor you will require Solar Cells.
We offer the following wafers for high quality Solar Cells:
FZ Si Item #
D445 $ Contact Us
100mm P/B (100) 380um SSP FZ 1-3 ohm-cm SEMI Prime, 1Flat, Epak cst, T>60µs
100mm Silicon wafers, per SEMI Prime, SSP 380±25µm, FZ P-type Si:B[100], Ro=(1-3)Ohmcm, One-side-polished, back-side Alkaline etched, SEMI Flat (one) TTV<10µm, Bow<20µm, Warp<30µm, Oxygen content <1E16/cc, Carbon<1E16/cc
These wafers are made from Float Zone crystallized electronic grade silicon. Unlike common solar cell wafers, these are essentially free of Oxygen, which prevents the formation of Boron-Oxygen complexes which lead to premature solar cell failure.
Reference #92234 for specs and pricing.
Silicon Based Photodiodes
A university researcher requested a quote for the following.
We are currently developing Silicon based photodiodes for Near Infrared wavelength detection.
We need a wafer from your company, for checking if optimal for our next prototype fabrication.
We need a wafer with these characteristics (see screenshot), it corresponds
Si Item #4017
100mm P/B (100) 1-10 ohm-cm 250um SSP Prime Grade
We would like to make tests if our technology (Silicon diodes with Near Infrared sensitivity up to 2.8µm) works with this wafer, hence it would be great if we can order 1 or 2 for trial.ice shipping option, maybe one unit fits in a smaller envelope?
Reference #312471 for specs and pricing.
Bonding Silicon Carbide to Fabricate Photodiodes
A corporate researcher requested a quote for the following.
We are looking to wafer bond these together build a photodiode. I seen on the attached excel file university wafer has available:
4H-N 3" dia,
Type/ Dopant : N / Nitrogen
Orientation :4 degree+/-0.5 degree
Thickness : 350 ± 25 um
B Grade,MPD<30 cm-2
B Grade,RT:0.01-0.1Ω·cm
B Grade,Bow/Warp/TTV<35um
Double face polished/Si face epi-ready with CMP,Surface Roughness : <0.5 nm
Reference #167250 for specs and pricing.
What Is A Photodiode?
A photodiode is a semiconductor device that converts light into an electrical current. It operates by allowing photons (light particles) to hit the photodiode's semiconductor material (often silicon or germanium), generating electron-hole pairs. This process creates a current directly proportional to the intensity of the incoming light.
Photodiodes are widely used in applications requiring precise light detection, such as: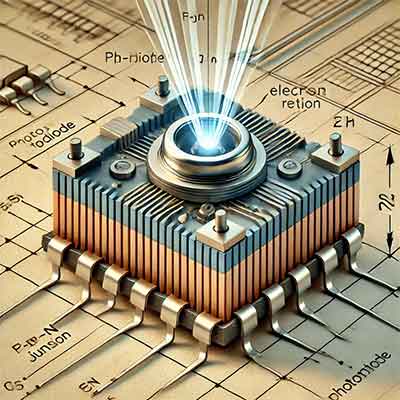
- Optical communication systems: Converting light signals into electrical signals.
- Photometry and radiometry: Measuring light intensity in scientific instruments.
- Solar cells: A specific type of large photodiode used to generate power.
- Safety and sensing: Detecting motion or presence through light interruption or reflection.
There are different types of photodiodes, such as PIN diodes and avalanche photodiodes, each suited to specific applications based on their sensitivity, response time, and noise characteristics.
What Substrates Are Used To Fabricate Photodiodes?
Photodiodes are typically fabricated on a variety of semiconductor substrates, each chosen based on its electronic properties, wavelength sensitivity, and application needs. Common substrates for photodiode fabrication include:
-
Silicon (Si):
- The most widely used substrate for visible and near-infrared (IR) detection (up to about 1,100 nm).
- Readily available and cost-effective with mature processing techniques.
- Suitable for applications like photometry, visible light sensors, and consumer electronics.
-
Germanium (Ge):
- Sensitive to a wider range in the near-infrared (up to about 1,800 nm).
- Often used in optical fiber communications and infrared sensing.
- Less commonly used due to higher costs and processing complexity.
-
Gallium Arsenide (GaAs):
- Primarily used in high-speed and high-frequency applications.
- Has high electron mobility and can detect wavelengths up to about 850 nm.
- Common in optical communications and military applications.
-
Indium Gallium Arsenide (InGaAs):
- Used for infrared photodetectors, extending detection up to 2,600 nm.
- Popular for telecommunications and spectroscopy due to its high sensitivity in the IR range.
- More expensive but offers low noise and fast response times.
-
Indium Phosphide (InP):
- Common in telecom applications for wavelengths around 1,550 nm, often paired with InGaAs.
- It has high electron mobility, good thermal stability, and is used for high-speed photodiodes.
-
Cadmium Telluride (CdTe) and Cadmium Zinc Telluride (CdZnTe):
- Used for X-ray and gamma-ray detection.
- High atomic numbers in these materials help improve sensitivity to higher-energy photons.
- Typically applied in medical imaging, astrophysics, and security screening.
-
Silicon Carbide (SiC) and Gallium Nitride (GaN):
- Suitable for ultraviolet (UV) detection.
- Both materials are radiation-hard and stable at high temperatures, making them ideal for space applications and harsh environments.
The choice of substrate depends on the specific application requirements for wavelength sensitivity, speed, noise performance, and cost.
Silicon Substrates Used To Fabricate Low Noise Photodiode Structure
A scientist researching high-energy physics requested a quote for the following.
I believe we want the following N+/N/P
silicon substrate: 4"Øx525μm, p-Si:B[100], P/E, Resistivity < 0.01 Ohmcm
n-Si epilayer, thick > 10 um, Resistivity > 10 Ohm cm
n+Si epilayer, thick about 5 um, Resistivity = 0.4 Ohm cm
Hopefully I have specified this correctly. We would like a lot of 25 wafers.
Do you have anything in stock that is close? If not, it would be great to get an idea about pricing and lead time for a custom order.
Also, are there any other specifications we should be using? This is for a low noise photodiode structure, so we want the highest quality material available.
UniversityWafer, Inc. Quoted:
Item Qty. Description
FG09. 9/18/25 Epi wafers, P/E 4"Ø×525±25µm, SEMI Flats (two),
Substrate: p-type Si:B[100]±0.5°, Ro=(0.010-0.020)Ohmcm,
One-side-polished, back-side Alkaline etched
EPI Layer: >5µm thick, n-type Si:P, Ro=(0.060-0.080)Ohmcm,
Reference #228512 for specs and pricing.
Hetero-Structure Grown Wafers Required for Photodiode Fabrication
A scientist researching space applications requested a quote for the following.
We have a requirement for specialized hetero-structure wafers that we plan to use in photodiode fabrication. We would appreciate it if you could provide a quote based on the specifications outlined below.
Specifications
We require two different epi-structures: InGaAs/InP and InGaAs/InAlAs, grown on 3-inch InP substrates using the MOCVD growth method. Detailed specifications for the substrate and epi-layer structures are attached for your reference. To summarize:
- Substrate: InP, 3 inches, semi-insulating, with the exact properties listed in Table-1.
- Epi-Structures: The wafers will include layers as per the specifications in Tables 2 and 3, covering material type, layer thickness, and doping concentrations.
- Application: These wafers will be used specifically for photodiode fabrication, with quality requirements as detailed below.
Quality and Testing Requirements
To ensure consistency, we need results from specific tests on each batch, including:
- Composition per layer (PL measurements for calibration).
- Carrier concentration (ECV or Hall measurements).
- Surface defect inspection using optical microscopy.
- Lattice matching and uniformity via XRD.
- Layer thickness confirmation using SEM or in-situ reflectance
Reference #276254 for specs and pricing.
How Do Photodiodes Work, and What Are They Used For?
Photodiodes work by converting light (photons) into an electrical current. When light hits the photodiode’s semiconductor material (like silicon or InGaAs), it creates electron-hole pairs in the material. Under an applied voltage or in a reverse-biased configuration, these electrons and holes move toward opposite terminals, generating a current proportional to the light's intensity. This current allows the photodiode to effectively measure the light's strength and frequency.
How Photodiodes Work
- Light Absorption: When photons enter the semiconductor, they generate electron-hole pairs.
- Separation of Charges: In reverse bias, an electric field across the depletion region of the p-n junction drives electrons and holes toward opposite terminals.
- Current Generation: The movement of these charges creates a measurable current, which is directly related to the light intensity.
Applications of Photodiodes
Photodiodes are widely used for:
- Optical Communications: Converting light signals in fiber optics to electrical signals.
- Light Sensing: In cameras, light meters, and ambient light sensors.
- Medical Equipment: In pulse oximeters, CT scanners, and other imaging devices.
- Industrial and Scientific Instruments: For accurate light measurements, including spectroscopy and photometry.
- Safety and Detection Systems: Motion detectors, smoke detectors, and proximity sensors.
Overall, photodiodes are essential for any application requiring precise, fast, and reliable light detection.
What is the difference between a photodiode and a solar cell?
The primary difference between a photodiode and a solar cell lies in their design focus and applications, even though both devices convert light into electrical energy.
1. Purpose and Application
- Photodiode: Primarily designed to detect light and generate a small current that corresponds to the light intensity. Used in light sensors, optical communication, and measurement devices.
- Solar Cell: Specifically optimized to convert sunlight into electrical power on a larger scale, with maximum efficiency. Used for energy generation in solar panels.
2. Operation Mode
- Photodiode: Usually operates in reverse bias (or sometimes zero bias) to improve speed and linearity for detecting light intensity. The reverse bias helps reduce response time and allows for quick detection of light changes.
- Solar Cell: Operates in zero bias (unbiased mode) to maximize energy output from sunlight. It is typically connected to a load to harness generated current efficiently.
3. Current and Power Output
- Photodiode: Generates a small current proportional to light intensity, typically in the microampere range, not optimized for power generation.
- Solar Cell: Designed to produce a higher current and power output, capable of driving a load, and optimized for maximum light-to-electricity conversion efficiency.
4. Design and Efficiency Optimization
- Photodiode: Prioritizes fast response times and linearity over high current output. It may have special structures like PIN or avalanche configurations for enhanced detection capabilities.
- Solar Cell: Optimized for energy conversion efficiency, with large surface areas to capture maximum light and additional layers to increase photon absorption.
In summary, while both devices convert light into electricity, photodiodes focus on precise light detection for sensor applications, whereas solar cells focus on high-efficiency energy conversion to generate power.
How does a photodiode differ from a phototransistor?
A photodiode and a phototransistor both detect light, but they differ in structure, operation, sensitivity, and applications. Here’s a comparison:
1. Structure and Components
- Photodiode: A simple semiconductor device with a p-n junction, which generates a small current directly proportional to light intensity.
- Phototransistor: A transistor with a light-sensitive base region. It amplifies the current generated by incoming light, resulting in a larger output signal.
2. Operation
- Photodiode: Operates mainly in reverse bias or zero bias. When photons hit the photodiode, they generate electron-hole pairs, creating a small current flow.
- Phototransistor: Functions similarly to a regular transistor but relies on light instead of an electrical current to trigger the base. When light strikes the base, it generates a current which is amplified by the transistor, leading to a stronger output.
3. Sensitivity and Speed
- Photodiode: Typically has a fast response time, making it ideal for high-speed applications where quick light detection is essential, such as optical communication.
- Phototransistor: More sensitive than a photodiode due to its amplification effect but generally slower in response. It’s suitable for applications where higher sensitivity is more important than speed, such as in light detection circuits.
4. Output Signal
- Photodiode: Produces a small current that is proportional to the light intensity without amplification.
- Phototransistor: Amplifies the small current from light exposure, resulting in a much larger output signal.
5. Applications
- Photodiode: Used in high-speed, precise light detection applications like fiber optics, optical sensors, and medical imaging.
- Phototransistor: Common in applications requiring higher sensitivity and moderate response times, such as light meters, IR receivers, and proximity sensors.
In summary, photodiodes are faster and provide a direct current proportional to light intensity, while phototransistors offer higher sensitivity and amplify the detected light signal but with a slower response time.
What are the main types of photodiodes, and how do they differ?
The main types of photodiodes include PN photodiodes, PIN photodiodes, avalanche photodiodes, and Schottky photodiodes. Each type has unique characteristics that make it suited for different applications. Here’s an overview of each:
1. PN Photodiode
- Structure: Simple p-n junction diode that generates a small current when exposed to light.
- Operation: Typically operates in reverse bias for enhanced sensitivity.
- Characteristics: Low cost, moderate sensitivity, and relatively low speed.
- Applications: Basic light detection, such as in solar cells and simple light sensors.
2. PIN Photodiode
- Structure: Contains an intrinsic (undoped) layer between the p-type and n-type regions, creating a wider depletion region.
- Operation: Enhanced sensitivity and faster response due to the intrinsic layer, which increases the photodiode’s active volume.
- Characteristics: Higher speed and sensitivity than standard PN photodiodes.
- Applications: Optical communication, high-speed detectors, and applications requiring fast response times.
3. Avalanche Photodiode (APD)
- Structure: Similar to a PIN photodiode but operated at high reverse bias voltages to achieve avalanche multiplication.
- Operation: In reverse bias, light-generated electron-hole pairs are multiplied through an avalanche effect, resulting in internal gain and amplified current.
- Characteristics: Extremely sensitive and capable of detecting very low light levels, but requires high voltage and has higher noise.
- Applications: Low-light detection, LIDAR, medical imaging, and applications where high sensitivity is essential.
4. Schottky Photodiode
- Structure: Comprises a metal-semiconductor junction (Schottky barrier) rather than a p-n junction.
- Operation: Detects light with fast response due to its small junction capacitance.
- Characteristics: Very high-speed response but generally less sensitive than PIN or avalanche photodiodes.
- Applications: High-speed applications like microwave and radio-frequency detection.
5. Other Specialized Photodiodes
- Quantum Photodiodes: Made from materials like InGaAs or HgCdTe for high sensitivity in specific wavelengths, often in the infrared range.
- Solar Cells: Technically a type of photodiode, optimized to convert light into electrical power rather than signal detection.
Summary of Differences:
- PN vs. PIN: PIN photodiodes are faster and more sensitive due to the intrinsic layer.
- Avalanche vs. PIN: Avalanche photodiodes offer higher sensitivity due to internal gain but require high bias voltage and have more noise.
- Schottky vs. PIN: Schottky photodiodes respond faster but are less sensitive, mainly used for ultra-high-speed applications.
In short, the choice of photodiode depends on the requirements for sensitivity, speed, wavelength range, and application.
What materials are used to make photodiodes, and why?
Photodiodes are made from various semiconductor materials, each chosen for its sensitivity to specific wavelengths and its electrical properties. Here are some commonly used materials and the reasons they are selected:
1. Silicon (Si)
- Wavelength Sensitivity: Visible to near-infrared light (up to ~1,100 nm).
- Properties: Silicon is readily available, cost-effective, and has well-established fabrication processes.
- Applications: Ideal for visible-light photodiodes, common in cameras, light meters, and consumer electronics.
2. Germanium (Ge)
- Wavelength Sensitivity: Extends further into the infrared (up to ~1,800 nm).
- Properties: Germanium has a lower bandgap than silicon, allowing it to detect longer wavelengths.
- Applications: Often used in infrared sensors and optical fiber communications.
3. Gallium Arsenide (GaAs)
- Wavelength Sensitivity: Visible to near-infrared.
- Properties: High electron mobility and radiation resistance make GaAs ideal for high-speed and high-frequency applications.
- Applications: Optical communication, high-speed photodiodes, and military applications.
4. Indium Gallium Arsenide (InGaAs)
- Wavelength Sensitivity: Near-infrared to mid-infrared (900 nm to ~2,600 nm).
- Properties: InGaAs has a tunable bandgap and high sensitivity in the infrared range, with low noise and high sensitivity.
- Applications: Telecommunications, spectroscopy, and night-vision equipment.
5. Indium Phosphide (InP)
- Wavelength Sensitivity: Often used as a substrate for InGaAs photodiodes.
- Properties: High electron mobility and good thermal stability.
- Applications: High-speed photodiodes for telecommunications, especially at wavelengths around 1,550 nm.
6. Cadmium Telluride (CdTe) and Cadmium Zinc Telluride (CdZnTe)
- Wavelength Sensitivity: X-ray and gamma-ray.
- Properties: High atomic numbers of cadmium and tellurium increase absorption efficiency for high-energy photons.
- Applications: Medical imaging, astrophysics, and security scanning.
7. Silicon Carbide (SiC) and Gallium Nitride (GaN)
- Wavelength Sensitivity: Ultraviolet (UV).
- Properties: Both materials are UV-sensitive and are radiation-hard, making them stable in harsh environments.
- Applications: UV detection in space applications, flame detection, and environmental monitoring.
Summary
Each material is chosen based on its bandgap and sensitivity to specific wavelengths. Silicon and InGaAs are the most common due to their performance and versatility across the visible and infrared spectrum. More specialized materials like CdTe and SiC cater to unique applications requiring high-energy photon detection or ultraviolet sensitivity.
What is the difference between PIN and avalanche photodiodes?
The primary difference between PIN and avalanche photodiodes (APDs) lies in their structure, operating principle, and performance characteristics, particularly in sensitivity and gain. Here’s a closer look:
1. Structure
- PIN Photodiode: Has three layers—p-type, intrinsic (undoped), and n-type—forming a p-i-n structure. The intrinsic layer widens the depletion region, allowing for efficient light absorption and charge separation.
- Avalanche Photodiode (APD): Similar to a PIN photodiode but operates under high reverse-bias voltage, enabling the avalanche effect in the depletion region, where incoming photons trigger a chain reaction of electron-hole pair generation.
2. Operating Principle
- PIN Photodiode: Photons hitting the intrinsic region create electron-hole pairs, which are quickly swept by the electric field to generate current. It operates with little or no internal amplification.
- APD: Under high reverse bias, the electron-hole pairs generated by light absorption undergo impact ionization, multiplying the carriers as they travel through the high electric field region. This avalanche effect amplifies the current, increasing the output signal.
3. Gain and Sensitivity
- PIN Photodiode: Has no internal gain; the current generated is directly proportional to the number of photons absorbed. It’s less sensitive than an APD but has lower noise.
- APD: Provides internal gain due to the avalanche multiplication, making it much more sensitive than a PIN photodiode. This makes APDs suitable for detecting low light levels. However, this gain introduces more noise.
4. Speed and Response Time
- PIN Photodiode: Generally faster with a quick response time due to a simpler structure and absence of gain, making it ideal for high-speed applications.
- APD: Slower than a PIN photodiode because of the multiplication process, though newer APD designs have improved speed.
5. Voltage Requirements
- PIN Photodiode: Operates at a lower reverse-bias voltage, which is easier to achieve and control.
- APD: Requires a high reverse-bias voltage to initiate the avalanche effect, which can be more challenging to manage and demands precise control for stable operation.
6. Applications
- PIN Photodiode: Used in high-speed applications like fiber-optic communication, bar code readers, and general light detection where moderate sensitivity suffices.
- APD: Ideal for low-light or long-distance applications requiring high sensitivity, such as LIDAR, medical imaging, and optical rangefinders.
Summary
In essence, PIN photodiodes are simpler, faster, and used where moderate sensitivity suffices, while APDs provide higher sensitivity through internal gain but with added noise and slower response, making them ideal for low-light and high-sensitivity applications.
How does reverse bias affect the operation of a photodiode?
Reverse bias plays a crucial role in enhancing the performance of a photodiode. Here’s how reverse bias impacts its operation:
1. Increases Depletion Region Width
- Applying reverse bias widens the depletion region of the photodiode. This expanded region allows for more efficient collection of electron-hole pairs generated by incoming photons, improving the photodiode’s sensitivity to light.
2. Reduces Capacitance
- Reverse bias reduces the capacitance of the photodiode, allowing it to respond more quickly to changes in light intensity. This lower capacitance makes the photodiode faster and better suited for high-speed applications, such as optical communication.
3. Improves Linearity
- In reverse bias, the current generated by the photodiode is more directly proportional to the light intensity. This linearity ensures that the output current accurately represents the amount of light hitting the photodiode, which is important for precise light measurement.
4. Increases Dark Current
- A drawback of reverse bias is an increase in “dark current,” which is the small current that flows through the photodiode even in the absence of light. While this dark current introduces noise, it is often minimal in quality photodiodes and can be managed in low-noise designs.
5. Enables Avalanche Multiplication (for APDs)
- For avalanche photodiodes (APDs), reverse bias is essential for the “avalanche effect.” At high reverse-bias voltages, the electric field is strong enough to cause impact ionization, amplifying the current produced by incoming photons. This process increases sensitivity, enabling APDs to detect very low light levels.
Summary
In short, reverse bias enhances a photodiode’s sensitivity, response speed, and linearity by expanding the depletion region and reducing capacitance. It’s essential for fast and accurate light detection, though it can also introduce some noise (dark current). For APDs, high reverse bias is necessary to enable the avalanche effect, providing significant amplification for low-light detection.
What applications benefit from using InGaAs or InP photodiodes?
InGaAs (Indium Gallium Arsenide) and InP (Indium Phosphide) photodiodes are highly sensitive to infrared light and are commonly used in applications that require detection of wavelengths beyond the visible spectrum. Their unique properties make them ideal for a variety of specialized applications:
1. Telecommunications and Fiber Optics
- InGaAs/InP photodiodes are widely used in fiber-optic communication systems due to their sensitivity in the near-infrared range (around 1,310 nm and 1,550 nm), which are common wavelengths for data transmission.
- They offer high-speed response and low noise, making them ideal for high-bandwidth data applications.
2. LIDAR (Light Detection and Ranging)
- InGaAs photodiodes, particularly in avalanche configurations, are used in LIDAR for long-range distance measurements and mapping. Their sensitivity in the near-infrared range enables LIDAR to function even in low-light conditions.
- Applications include autonomous vehicles, environmental monitoring, and topographic mapping.
3. Spectroscopy
- InGaAs photodiodes are popular in near-infrared (NIR) spectroscopy for chemical and molecular analysis, as they can detect NIR wavelengths that are sensitive to organic compounds.
- Applications include medical diagnostics, pharmaceutical analysis, food quality testing, and environmental sensing.
4. Thermal Imaging and Night Vision
- InGaAs photodiodes are useful for thermal imaging in the short-wave infrared (SWIR) range, offering a way to detect heat signatures and other low-light phenomena.
- Used in night-vision systems, security, military applications, and industrial inspection.
5. Astronomy and Space Applications
- InGaAs/InP photodiodes are sensitive enough for astrophotography and satellite imaging in the NIR range, which helps in observing distant stars and galaxies or in detecting IR radiation from astronomical objects.
- InP’s radiation hardness also makes it suitable for spaceborne sensors and photodetectors, which endure harsh cosmic environments.
6. Environmental Monitoring and Gas Sensing
- These photodiodes are sensitive to specific NIR wavelengths absorbed by gases, allowing for the detection of pollutants like CO₂, methane, and other hydrocarbons.
- Applications include air quality monitoring, industrial safety, and greenhouse gas tracking.
7. Biomedical Imaging
- InGaAs photodiodes are used in optical coherence tomography (OCT) and other imaging techniques for tissue analysis, eye imaging, and diagnostics, taking advantage of their sensitivity in deeper tissue-penetrating wavelengths (NIR).
8. Laser Range Finding and Targeting
- InGaAs/InP photodiodes are also applied in laser range finders for military and industrial purposes. Their high-speed response and sensitivity to laser light in the NIR range make them ideal for accurate distance measurement and object tracking.
Summary
InGaAs and InP photodiodes are vital in applications that require high sensitivity and precision in the near-infrared range, including telecommunications, LIDAR, spectroscopy, thermal imaging, astronomy, and biomedical imaging. Their properties make them irreplaceable for tasks needing low-light or high-speed IR detection.
How do photodiode response time and sensitivity work?
Photodiode response time and sensitivity are critical performance parameters that define how well a photodiode detects and reacts to light signals. Here’s how they work and what factors affect them:
1. Response Time
-
Definition: The response time of a photodiode is the speed at which it can detect and respond to changes in light intensity. It includes both the rise time (how quickly the photodiode responds to an increase in light) and fall time (how quickly it recovers after the light source is removed).
-
Influencing Factors:
- Depletion Region Width: A wider depletion region allows faster collection of electron-hole pairs, reducing response time. PIN photodiodes, which have an intrinsic (undoped) layer, often have a wider depletion region and therefore faster response times.
- Capacitance: Lower capacitance allows for faster switching times, making the photodiode respond more quickly to rapid changes in light. Reverse biasing reduces capacitance, enhancing response time.
- Carrier Transit Time: The time it takes for carriers (electrons and holes) to travel through the depletion region affects response time. Higher electric fields (achieved by reverse biasing) can accelerate carriers, reducing transit time and enabling faster response.
-
Applications with High Response Time Needs: High-speed photodiodes, such as those in optical communication or high-speed sensors, rely on very short response times to quickly detect rapid light fluctuations in data signals.
2. Sensitivity
-
Definition: Sensitivity refers to a photodiode's ability to detect low levels of light and convert them into a measurable current. High sensitivity means the photodiode can generate a larger current for a given amount of light.
-
Influencing Factors:
- Material Choice: The semiconductor material determines the photodiode’s sensitivity range and wavelength response. For example, silicon is sensitive to visible and near-infrared light, while InGaAs photodiodes are more sensitive in the infrared range.
- Reverse Bias: Applying reverse bias increases sensitivity by expanding the depletion region, allowing more light-generated carriers to be collected efficiently.
- Internal Gain (Avalanche Effect): In avalanche photodiodes (APDs), a high reverse bias creates an “avalanche” of carriers, amplifying the signal. This internal gain significantly increases sensitivity, allowing APDs to detect very low light levels.
- Noise Characteristics: Lower noise levels improve effective sensitivity, as noise can obscure faint signals. APDs are more sensitive but can also introduce more noise, so sensitivity is a balance between amplification and noise management.
-
Applications with High Sensitivity Needs: Low-light applications, such as night vision, spectroscopy, and medical imaging, require photodiodes with high sensitivity to detect weak light signals.
Summary
- Response Time determines how quickly the photodiode reacts to changing light signals and is crucial for high-speed applications.
- Sensitivity is about detecting low light levels and generating a sufficient electrical signal in response, which is essential for applications that operate in low-light conditions.
In short, faster response time is achieved through design choices that speed up carrier collection, while higher sensitivity is achieved by maximizing light collection and signal amplification.
What factors affect the noise and efficiency of photodiodes?
The noise and efficiency of photodiodes are influenced by various factors related to their design, operating conditions, and material properties. Here’s a closer look at the key factors affecting each:
Factors Affecting Noise in Photodiodes
-
Dark Current
- Description: Dark current is the small leakage current that flows through a photodiode even when no light is present. It’s a primary source of noise, especially in low-light applications.
- Influence: Higher temperatures increase dark current, which in turn increases noise. Minimizing dark current through cooler operating conditions or using materials with lower leakage rates (e.g., silicon) reduces noise.
-
Thermal Noise
- Description: Also known as Johnson or Nyquist noise, thermal noise is generated by the random motion of charge carriers due to temperature.
- Influence: Thermal noise increases with temperature and can be minimized by reducing the resistance in the photodiode circuit or cooling the device.
-
Shot Noise
- Description: Shot noise arises from the discrete nature of current flow, especially in reverse bias conditions, and is proportional to the square root of the current.
- Influence: High bias current increases shot noise. Operating at lower reverse bias or using a photodiode with a lower dark current can help reduce shot noise.
-
1/f (Flicker) Noise
- Description: Flicker noise, or 1/f noise, is more pronounced at low frequencies and increases with factors like imperfections in the photodiode material or contact surfaces.
- Influence: Flicker noise can be minimized by using high-quality materials and maintaining clean, stable contacts, which is particularly important in high-sensitivity applications.
-
Excess Noise in Avalanche Photodiodes (APDs)
- Description: In APDs, the internal gain amplification process can add excess noise due to the randomness of carrier multiplication.
- Influence: The gain in APDs should be carefully managed to achieve a balance between high sensitivity and low noise. APDs typically require low-noise amplifiers and signal processing to manage this excess noise.
Factors Affecting Efficiency in Photodiodes
-
Material and Bandgap
- Description: The semiconductor material and its bandgap affect the range of wavelengths the photodiode can detect.
- Influence: Materials like silicon, InGaAs, and germanium are chosen based on the application’s required wavelength sensitivity. Using the right material for the target wavelength improves quantum efficiency, which is the photodiode’s ability to convert photons into charge carriers.
-
Quantum Efficiency (QE)
- Description: QE is the percentage of photons that are converted into electron-hole pairs. Higher QE translates to better efficiency.
- Influence: QE is influenced by factors like surface passivation, anti-reflective coatings, and the thickness of the depletion region. Thicker depletion regions and anti-reflective coatings help increase QE by improving light absorption and minimizing surface reflections.
-
Depletion Region Width
- Description: A wider depletion region allows more light to be absorbed and creates a stronger electric field to collect generated carriers.
- Influence: Reverse bias can be used to increase the depletion region width, improving efficiency, particularly for longer wavelengths.
-
Surface Reflectance
- Description: Surface reflectance refers to the amount of light reflected off the photodiode surface, which reduces the light that enters and is absorbed.
- Influence: Anti-reflective coatings on the photodiode surface reduce reflection and allow more photons to reach the active region, increasing efficiency.
-
Temperature Effects
- Description: Higher temperatures increase dark current and noise, which can reduce the effective efficiency of photodiodes.
- Influence: Operating photodiodes at lower temperatures helps maintain a high signal-to-noise ratio, effectively improving efficiency, especially in low-light conditions.
-
Reverse Bias Voltage
- Description: Reverse bias widens the depletion region, enhancing carrier collection and improving efficiency.
- Influence: Although reverse bias can increase efficiency, it must be carefully controlled to avoid excessive noise (especially in APDs).
Summary
- Noise is affected by factors such as dark current, thermal noise, shot noise, and excess noise in APDs. Managing temperature, using high-quality materials, and optimizing reverse bias can help reduce noise.
- Efficiency depends on the photodiode’s material, quantum efficiency, depletion region width, surface reflectance, temperature, and reverse bias voltage. Proper material choice, anti-reflective coatings, and optimized operating conditions help maximize efficiency.
A balance between low noise and high efficiency is essential for optimal photodiode performance, particularly in applications requiring high sensitivity and precise light detection.