Which Type of Silicon Wafer is Good for Photolithography?
A postdoc asked the following question:
Hi, can you tell me the difference between N-type and P-type silicon wafer? Which one is good for photolithograpy?
Answer:
P-type and n-type define the predominant type of electrical conduction. This is a key electronic parameter.
Both n-type and p-type wafers are equally good for photo-lithography.
Reference #208785 for specs and pricing.
Substrates Used for Customized Photolithography?
A Phd requested a quote for the following:
Do you provide services for customized photolithography and sell patterned silicon wafers with developed photoresists? This is a rough pattern. All the corners will be rounded. The unit is mm. The width of the lines would be 100 μm, and the desired height would be 50 μm. We would prefer SU-8 on Silicon.
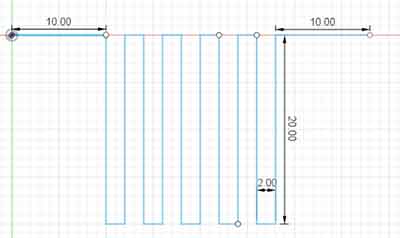
an I quote this for Chrome on glass or does this need to be on a wafer? If so we would image this on a 4”x4”x.060” Soda-Lime glass with chrome and then cut down.
Quantity: <10
Approximate area: 30 mm * 50 mm
Patterns in CAD
Reference #265648 for specs and pricing.
What Silicon Wafers are Used to Create Molds for PDMS Microfluidic Devices Using Photolithography?
A graduate student studying microfluidic devices requested a quote for the following:
I work at a lab and we use your wafers to create molds for PDMS microfluidic devices using photolithography. Do people usually use the 1196 mechanical grade silicon wafers wafer for molds for PDMS devices?
Answer: No, these are mechanical grade wafers and are sold as-is. You need either test grade or even prime grade to ensure the flattest cleanest polished silicon wafer surface.
Reference #237896 for specs and pricing.
What Ultra-Thin Silicon Wafers are Used for Photolithography?
A Phd candidate requested the following:
Question:
I am interested in thin Si wafer I found from webpage that you have large stock inventory of thin silicon wafers from 5um-50um, but could find it in online-inventory. Could you provide full inventory or product list for me to choose the most appropriate options. I am looking for Si wafer 2 or 3 inch-diameter with thickness from 5um to 100um. I am also interested in "Rigid wafer ring" for handing the thin wafers, but could not image how it looks like and how the wafer is attached. Do you have some more information on this and price as well? I do not have much constraints in those properties, butshould be <100>. Undoped or low-resistance is preferred but it depends on price as well.
Answer:
Actually, ultra-thin silicon wafers are rather strong. They handle like paper.
They will withstand a vacuum chuck that is meant for such wafers.
A 2"Ø wafer you can handle with a vacuum wand and even with teflon tweezers if you carry it vertically. If you do have to mount it, then use acetone soluble wax. Heat it, and the wafer sides off.
Reference #224131 for specs and pricing.
What Substrates are Used to Manufacture Diffractive Optics Through Photolithography?
I am a doctoral student looking to purchase fused silica wafers for manufacturing diffractive optics through photolithography. I know I would like my wafers to be 2 inches in diameter and 500 microns or less thick, but am unsure about type, dopant or grade. Could you help me in selecting the right choice?
Reference #255421 for specs and pricing.
How to Fabricate a Silicon Mesh for Atomizing Liquid
A lab researcher requested help sourcing substrates for their research:
Question:
We are looking for a 75 micrometer thick <100> undoped Si wafer (4 inch). Is it possible to purchase 75 micrometer thick wafers? I hope it is not too hard to handle because it's too thin.
I attached a short slide of what we are trying to make.
In the end, we want to make a thin silicon mesh with cone-shaped holes (10 - 50 micrometers).
The plans are
- Deposit SiO2 through PECVD
- Apply photoresist and create a pattern through photolithography
- Dip it in HF for creating pattern on SiO2 hard mask
- Remove the photoresist
- Dip it in KOH to create holes in the Si wafer
- Dip it in HF to remove the SiO2 hard mask
This is our first time working on Si wafers, so I'm not really sure if the steps will even work. Please let us know what you think and whether this is possible with one of your wafers.
Answer:
Almost every one of the Silicon wafers that we carry is suitable for doing what you describe.
We can also supply such wafers with an Oxide layer, either Thermal Oxide or SiO2 deposited by PECVD. Thermal Oxide is dense and essentially monocrystalline, and it grows on both sides of the silicon wafer, both on polished and unpolished sides
PECVD applied Oxide is also dense but more glass-like, and it is normally applied to the polished wafer side only.
If you want to etch holes in a Silicon wafer then I am unsure why you need the Oxide layer. The hole pattern is created out of the photoresist and holes are etched through it. However I do not think that it is practical to etch holes 50µm in diameter and 380µm deep.
Still, we can supply whatever Silicon wafers that you specify and apply SiO2 or other coatings to them as you require.
Reference #256474 for specs and pricing.
What is Photolithography?
Photolithography is one of the most popular microfabrication techniques for creating features on a photo-sensitive chemical, called a photoresist, spin coated over a substrate (usually silicon or glass).
It utilizes optical means to transfer a user-defined pattern from a photomask to the photoresist by exposing it to ultraviolet light. Usually, it is used to pattern semiconductor devices.
It is a method of patterning
Photolithography is one of the most widely used methods of microfabrication. It utilizes a light-sensitive polymer called a photoresist that is spin coated onto a substrate (usually silicon or glass) and then exposed to UV light in order to transfer a user-defined pattern.
It is one of the most important techniques for creating extremely detailed patterns on a surface of a material, such as a semiconductor wafer or a chip. It can produce patterns as small as tens of nanometers in size. It can also be used to create complex patterns over an entire wafer, and it has many advantages over other methods of patterning.
Lithography has been around since 1796, but only in the last 50 years has it become a viable technique for making chips and other small devices. The main advantage of photolithography is that it provides exact control over the shape and size of the objects that it creates, which is important for designing integrated circuits and other complex electronics.
In order to achieve this, the substrate must first be prepared in a series of controlled processes. These include substrate cleaning, spin coating of the photoresist, soft baking of the resist and exposure to UV light through a photomask that is usually made from quartz coated with an opaque material like chromium.
The most important aspect of the lithography process is the alignment of the photomask with the substrate. This is often done using an expensive and complicated machinery called a'mask aligner'.
Another important factor is the thickness of the photoresist. The thickness of the resist is related to the wavelength of the light that is used in the exposure and it helps to improve the resolution. In addition, the wavelength can be increased or decreased by optimizing the intensity of the light.
In the past, the most common source of light for lithography has been gas-discharge lamps that use mercury and noble gases to emit ultraviolet (UV) radiation. This ultraviolet (UV) light is usually at a wavelength of 193 nm or higher, which is the maximum allowed for insulating materials such as silicon dioxide.
It is a method of printing
Photolithography is a microfabrication process that uses optical means to transfer a user-defined pattern to a substrate material. It is one of the most widely used processes in producing feature-level micro- and nanoscale features on a surface.
It utilizes a photo-sensitive polymer, called photoresist, that loses its resistance or susceptibility to attack when it is exposed to light. A mask with a desired feature is placed above the photoresist and illuminated to expose the pattern in the resist.
After exposure, the resist is immersed in a chemical developer (solvent). The developer dissolves away areas of the resist that have been exposed to light. The result is either a positive or negative resist pattern depending on the type of radiation and the nature of the resist.
In addition to photolithography, there are other methods of printing such as X-ray lithography and ion beam lithography. In the semiconductor industry, these methods are used to produce chips on silicon wafers.
However, a new type of lithography technique is making it possible to print microchips on silicon wafers at lower cost and with higher throughput than traditional lamp-based techniques. This new technique uses ultraviolet (UV) light, which is typically 365 nm in wavelength. This wavelength is able to penetrate the glass photolithography mask and reach the silicon substrate beneath it.
Another important advantage of this method is that it allows for precise control over the depth, shape and overall size of the pattern. This gives the designer much more control over the final product and is especially useful for nanotechnology, where precision is critical.
The resolution of photolithography depends on the light wavelength, thickness of the resist and the k factor. A k factor of 0.4 is a typical value for production. This enables features to be created as small as 16 nm. It is also possible to decrease the k factor by changing the intensity of the light.
In the past, lithography was performed using mercury lamps. These lamps produced light across a broad spectrum, with strong peaks in the UV range. These lamp-based techniques are no longer adequate for high-end applications in the semiconductor industry, so a new type of lithography is needed to meet the demands of the industry.
It is a method of semiconductor fabrication
Photolithography is the name given to a number of different methods used to transfer a pattern onto the surface of a substrate, usually a silicon wafer. This can be done by using a laser, an electron beam or an ion beam.
The most common form of lithography involves the use of a mask, which blocks some areas of light but allows others to pass. The mask is then positioned over the wafer so that a light beam can pass through it to project an image on the wafer. Other types of lithography include diamond patterning, which uses diamonds as a resist to transfer patterns to the wafer.
A layer of a chemical photoresist is then deposited on the silicon wafer, which changes its properties when exposed to light. The chemical photoresist is then removed, either by a liquid "resist stripper" or by plasma-like oxygen ions.
This is often the first step in a semiconductor fabrication process, which requires hundreds of sequential steps to make an IC (integrated circuit) device. Each layer in the process - which includes epitaxial growth/deposition, patterning and etching, implantation and diffusion, deposition of interconnection metals and chemical mechanical planarization - has to be fabricated to specific dimensions with very low defectivity.
Modern lithography tools are now capable of producing feature sizes as small as 50 nm in the deep ultraviolet range (DUV) from excimer lasers. This has enabled the Moore's Law trend of miniaturization in the industry to continue for more than 20 years.
As a result, a single chip contains hundreds of identical transistors that must be carefully manufactured and tested to ensure quality. The chips are then cut from the wafer and mounted on a plastic or metal package to be shipped.
Traditionally, the process involved mercury bulbs that produced spectral lines at 436 nm ("g-line") and 405 nm (“h-line”). However, these light sources had poor resolution, slow throughput and high cost, which led to the development of free-electron lasers.
In recent decades, lasers have become the standard light source for lithography tools, with wavelengths at 248 and 193 nm allowing for features that are smaller than 0.1 micrometer in width. These lasers also have very low energy loss, making them a good choice for large-area wafers.
It is a method of etching
Photolithography is a microfabrication technique used to create custom patterns on the surface of wafers. It is an important technique for manufacturing substrates for electronics, sensors, and microfluidic devices.
The basic principle of photolithography is to transfer copies of a master pattern onto the surface of a substrate, usually of some material (such as silicon wafers). This is done by coating a thin layer of a polymer known as a photoresist, which is sensitive to ultraviolet light, and then exposing it to light. The photoresist subsequently undergoes a series of chemical, physical, and optical processes to form the final micro-structured pattern on the wafer’s surface.
For example, photolithography can be used to create a pattern of holes on a semiconductor wafer that will be used for ion implantation or for other patterning techniques such as wet chemical etching and plasma etching. It is also used to open areas on the surface of a substrate for the growth of new layers by chemical plating, for example in copper interconnects.
It is also used to create a pattern of eliminations, or the removal of a certain amount of material, from a sample before the next process step occurs that will affect the entire sample. The pattern of eliminating materials is called the etch mask, and it is usually made by exposing a glass or fused silica substrate with a Chromium etch to a layer of photoresist.
When the etching process is complete, the resist layer will be stripped, leaving the desired pattern etched into the deposited layer. The etching process can be either wet or dry, depending on the type of resist used.
Optical lithography is the most common type of lithography, but electron beam lithography and diamond patterning are other methods that can be used to create micro-structured patterns on substrates. E-beam lithography is faster and more efficient than optical lithography but is less precise in the patterning of the wafer's surface.
Optical lithography is the most popular method of creating micro-structured patterns on substrates, and it can be performed in both conventional and high resolution. The primary limiting factor in optical lithography is the wavelength of the light used, which is typically ultraviolet or deep ultraviolet. However, with the development of free-electron lasers and other alternative light sources, wavelengths have continued to decrease, to extreme ultraviolet or X-ray.
Video: Photolithography: Step-by-Step
What is Lithography?
Lithography is a process of creating a pattern on a surface by selectively exposing it to light, typically using a mask or template to define the pattern. The term "lithography" comes from the Greek words "lithos" (stone) and "graphein" (to write), reflecting the historical use of stone plates in the process.
There are several types of lithography, but the most common method used in modern technology is photolithography. In photolithography, a photosensitive material (such as a photoresist) is deposited on a substrate (such as a silicon wafer), and a mask is placed over the material to define the pattern to be created. The mask is then illuminated with a light source (such as ultraviolet light), which causes the exposed areas of the photosensitive material to undergo a chemical change. The unexposed areas are then removed, leaving behind the desired pattern.
Photolithography is used extensively in the semiconductor industry to create the complex patterns of circuits and components on silicon wafers used in electronic devices. It is also used in the production of microelectromechanical systems (MEMS), microfluidic devices, and other precision components. Other types of lithography include electron beam lithography, X-ray lithography, and ion beam lithography, which are used for creating even smaller features than those achievable with photolithography.